|
 |
 |
 |
 |
|
|




Neurotoxicity

The nervous system is very complex and toxins can act at many different points in this complex system. This section can not possibly describe in detail the
anatomy and physiology of the nervous system. Neither can it provide in-depth information on the many neurotoxins in our environment and the subtle ways they can damage the nervous system or interfere with its function. Since the nervous system innervates all areas of the body, some toxic effects may be quite specific and others very generalized depending upon where in the nervous system the toxin exerts its effect. Before discussing how neurotoxins cause damage lets look at the basic
anatomy and physiology of the nervous system
Anatomy and Physiology of the Nervous System

The nervous system has three basic functions. First, specialized cells detect sensory information from the environment and relay that information to other parts of the nervous system. Second, it directs motor functions of the body, usually in response to sensory input. Finally, part of the nervous system is involved in processing of information. The third function is to integrate the thought processes, learning, and memory. All of these functions are potentially vulnerable to the actions of toxicants.
The nervous system consists of two fundamental anatomical divisions:
 |
 |
 |
|  | Central nervous system (CNS)
|
 |
|  | Peripheral nervous system (PNS).
|
 |
The CNS includes the brain and spinal cord. The CNS serves as the control center and processes and analyzes information received from sensory receptors and in response issues motor commands to control body functions. The brain, which is the most complex organ of the body, structurally consists of six primary areas:
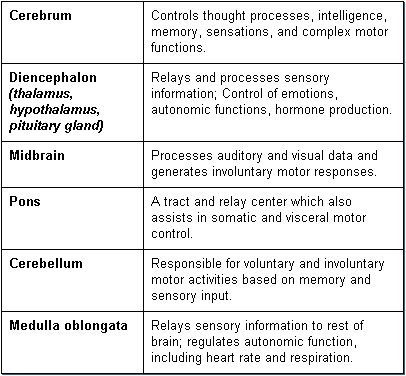
 |
 |
 |
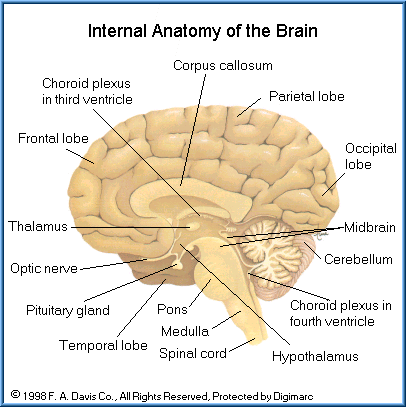 |
|
 V. C. Scanlon and T. Sanders, Essentials of
Anatomy and Physiology, 2nd edition. F. A. Davis, 1995. |
|
The PNS consists of all nervous tissue outside the CNS. The PNS contains two forms of nerves: afferent nerves, which relay sensory information to the CNS, and efferent nerves, which relay motor commands from the CNS to various muscles and glands.
Efferent nerves are organized into two systems. One is the somatic nervous system (also known as the voluntary system) which carries motor information to skeletal muscles. The second efferent system is the autonomic nervous system, which carries motor information to smooth muscles, cardiac muscle, and various glands. The major difference in these two systems is one of conscious control. The somatic system is under our voluntary control (for example, we can move our arms by consciously tell our muscles to contract). In contrast, we can not consciously control the smooth muscles of the intestine, heart muscle or secretion of hormones. Those functions are automatic and involuntary as controlled by the autonomic nervous system.
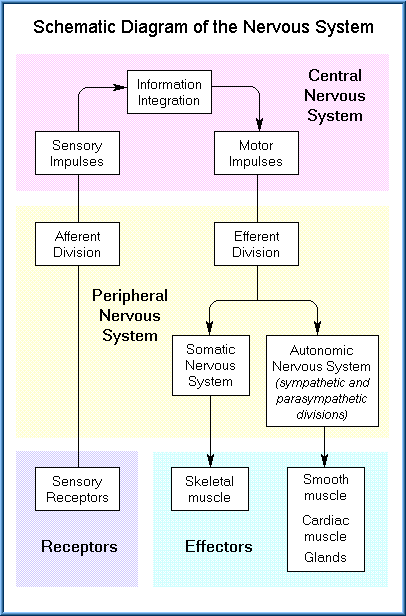
Cells of the Nervous System

Two categories of cells are found in the nervous system, neurons and glial cells. Neurons are the functional nerve cells directly responsible for transmission of information to and from the CNS to other areas of the body. Glial cells (also known as neuroglia) provide support to the neural tissue, regulate the environment around the neurons, and protect against foreign invaders.
Neurons communication with all areas of the body and are present within both the CNS and PNS. They serve to transmit rapid impulses to and from the brain and spinal cord to virtually all tissues and organs of the body. As such, they are a most essential cell and their damage or death can have critical effects on body function and survival. When neurons die, they are not replaced. As neurons are lost so are certain neural functions such as memory, ability to think, quick reactions, coordination, muscular strength, and our various senses (such as impaired sight, hearing, taste, etc.). If the neuron loss or impairment is substantial, severe and permanent disorders can occur, such as blindness, paralysis, and death.
A neuron consists of a cell body and two types of extensions, numerous dendrites, and a single axon. Dendrites are specialized to receive incoming information and send it to the neuron cell body with transmission (electrical charge) on down the axon to one or more junctions with other neurons or muscle cells (known as synapses). The axon may extend long distances, over a meter in some cases, to transmit information from one part of the body to another. Some axons are wrapped in a multi-layer coating, known as the myelin sheath, which helps insulate the axon from surrounding tissues and fluids and prevents the electrical charge from escaping from the axon.
 |
 |
 |
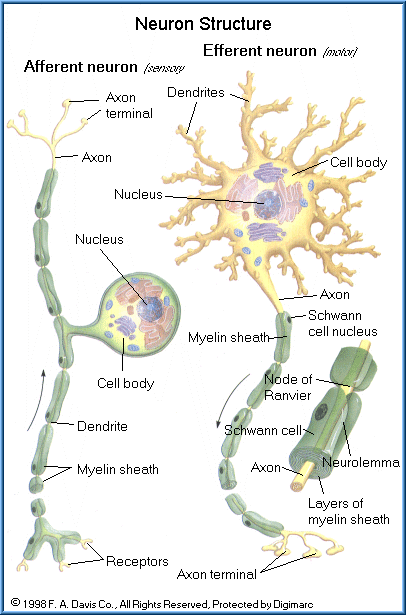 |
|
 V. C. Scanlon and T. Sanders, Essentials of
Anatomy and Physiology, 2nd edition. F. A. Davis, 1995. |
|
Information is passed along the network of neurons between the CNS and the sensory receptors and the effectors by a combination of electrical pulses and chemical neurotransmitters. The information (electrical charge) moves from the dendrites through the cell body and down the axon. The mechanism by which an electrical impulse moves down the neuron is quite complex. When the neuron is at rest, it has a negative internal electrical potential. This changes when a neurotransmitter binds to a dendrite receptor. Protein channels of the dendrite membrane open allowing the movement of charged chemicals across the membrane, which creates an electrical charge. The propagation of an electrical impulse (known as action potential) proceeds down the axon by a continuous series of opening and closing of sodium-potassium channels and pumps. The action potential moves like a wave from one end (dendritic end) to the terminal end of the axon.
However, the electrical charge can not cross the gap (synapse) between the axon of one neuron and the dendrite of another neuron or an axon and a connection with a muscle cell (neuromuscular junction). The information is moved across the synapse by chemicals (neurotransmitters).
Neurons do not make actual contact with one another but have a gap, known as a synapse. As the electrical pulse proceeds up or down an axon, it encounters at least one junction or synapse. An electrical pulse can not pass across the synapse. At the terminal end of an axon is a synaptic knob, which contains chemicals, known as neurotransmitters.
Neurotransmitters are released from vesicles upon stimulus by an impulse moving down the presynaptic neuron. The neurotransmitters diffuse across the synaptic junction and binds to receptors on the postsynaptic membrane. The neurotransmitter-receptor complex then initiates the generation of an impulse on the next neuron or the effector cell, e.g., muscle cell or secretory cell.
After the impulse has again been initiated, the neurotransmitter-complex must be inactivated or continuous impulses (above and beyond the original impulse) will be generated. This inactivation is performed by enzymes, which serve to break down the complex at precisely the right time and after the exact impulse has been generated. There are several types of neurotransmitters and corresponding inactivating enzymes. One of the major neurotransmitters is acetylcholine with acetylcholinesterase as the specific inactivator.
 |
 |
 |
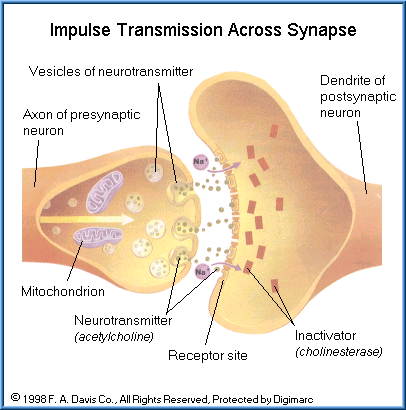 |
|
 V. C. Scanlon and T. Sanders, Essentials of
Anatomy and Physiology, 2nd edition. F. A. Davis, 1995. |
|
There are over 50 known neurotransmitters, although only the following are reasonably well understood.
 |
 |
 |
|  | Acetylcholine
|
 |
|  | Norepinepharine (noradrenaline)
|
 |
|  | Serotonin
|
 |
|  | Gamma aminobutyric acid (GAMA)
|
 |
Neurons are categorized by their function and consist of three types:
Sensory neurons (afferent neurons) carry information from sensory receptors (usually processes of the neuron) to the CNS. Some sensory receptors detect external changes such as temperature, pressure and the senses of touching and vision. Others monitor internal changes such as balance, muscle position, taste, deep pressure and pain.
Motor neurons (effector neurons) relay information from the CNS to other organs terminating at the effectors. Motor neurons make up the efferent neurons of both the somatic and autonomic nervous systems.
Interneurons (association neurons) are located only in the CNS and provide connections between sensory and motor neurons. They can carry either sensory or motor impulses. They are involved in spinal reflexes, analysis of sensory input, and coordination of motor impulses. They also play a major role in memory and the ability to think and learn.
 |
 |
 |
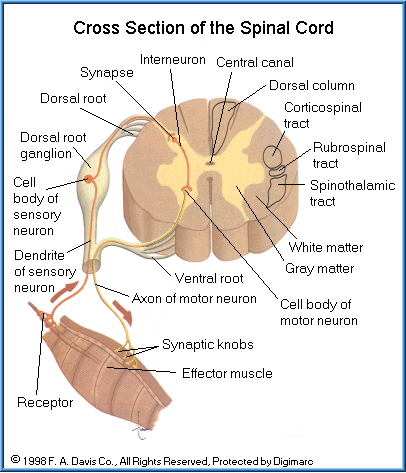 |
|
 V. C. Scanlon and T. Sanders, Essentials of
Anatomy and Physiology, 2nd edition. F. A. Davis, 1995. |
|
Glial cells are important as they provide a structure for the neurons (like an organized bed for them to lie in); they protect them from outside invading organisms, and maintain a favorable environment (nutrients, oxygen supply, etc.) for them. The neurons are highly specialized and do not have all the usual cellular organelles to provide them with the same life-support capability. They are highly dependent on the glial cells for their survival and function. For example, neurons have such a limited storage capacity for oxygen that they are extremely sensitive to decreases in oxygen (anoxia) and will die within a few minutes. The types of glial cells are described below:
Astrocytes are big cells, only in the CNS, and maintain the blood-brain barrier that controls the entry of fluid and substances from the circulatory system into the CNS. They also provide rigidity to the brain structure.
Schwann cells and oligodendrocytes wrap themselves around some axons to form myelin, which serves like insulation. Myelinated neurons usually transmit impulses at high speed, such as needed in motor neurons. Loss of myelination causes a dysfunction of these cells.
Microglia are small, mobile, phagocytic cells.
The CNS is bathed in a special fluid the cerebral spinal fluid (CSF), which is produced by the ependymal cells.
Toxic Damage to Nervous System

The nervous system is quite vulnerable to toxins since chemicals interacting with neurons can change the critical voltages, which must be carefully maintained. However, most of the CNS is protected by an anatomical barrier between the neurons and blood vessels, known as the blood-brain barrier.
The most significant changes consist of tighter junctions between endothelial cells of the blood vessels in the CNS and the presence of astrocytes surrounding the blood vessels. This prevents the diffusion of chemicals out of the blood vessels and into the intracellular fluid except for small, lipid-soluble, non-polar molecules. Specific transport mechanisms exist to transport essential nutrients (such as glucose and amino acids and ions) into the brain. Another defense mechanism within the brain to counter chemicals that pass through the vascular barrier is the presence of metabolizing enzymes. Certain detoxifying enzymes, such as monoamine oxidase, can biotransform many chemicals to less toxic forms as soon as they enter the intercellular fluid.
The basic types of changes due to toxins can be divided into three categories - sensory, motor and interneuronal - depending on the type of damage sustained.
Damage can occur to sensory receptors and sensory neurons, which can affect the basic senses of pressure, temperature, vision, hearing, taste, smell, touch, and pain. For example, heavy metal poisoning (especially lead and mercury) can cause deafness and loss of vision. Several chemicals including inorganic salts and organophosphorus compounds can cause a loss of sensory functions.
Damage to motor neurons can cause muscular weakness and paralysis. Isonicotinic hydrazide (used to treat tuberculosis) can cause such damage.
Interneuronal damage can cause learning deficiencies, loss of memory, incoordination, and emotional conditions. Low levels of inorganic mercury and carbon monoxide can cause depression and loss of memory.
Mechanisms for Toxic Damage to the Nervous System

Toxic damage to the nervous system occurs by the following basic mechanisms:
 |
 |
 |
|  | Direct damage and death of neurons and glial cells
|
 |
|  | Interference with electrical transmission
|
 |
|  | Interference with chemical neurotransmission
|
 |
Death of Neurons and Glial Cells: The most common cause of death of neurons and glial cells is anoxia, an inadequate oxygen supply to the cells or their inability to utilize oxygen. Anoxia may result from the bloods decreased ability to provide oxygen to the tissues (impaired hemoglobin or decreased circulation) or from the cells unable to utilize oxygen.
For example, carbon monoxide and sodium nitrite can bind to hemoglobin preventing the blood from being able to transport oxygen to the tissues. Hydrogen cyanide and hydrogen sulfide can penetrate the blood-brain barrier and is rapidly taken up by neurons and glial cells. Another example is fluoroacetate (commonly known as Compound 1080, a rodent pesticide) which inhibits a cellular enzyme. Those chemicals interfere with cellular metabolism and prevent nerve cells from being able to utilize oxygen. This type of anoxia is known as histoxic anoxia.
Neurons are among the most sensitive cells in the body to inadequate oxygenation. Lowered oxygen for only a few minutes is sufficient to cause irreparable changes leading to death of neurons.
Several other neurotoxins are known to directly damage or kill neurons, including:
 |
 |
 |
|  | lead
|
 |
|  | mercury
|
 |
|  | some halogenated industrial solvents, including methanol (wood alcohol)
|
 |
|  | glutamate (an amino acid in some food products)
|
 |
|  | trimethyltin, and
|
 |
|  | MPTP (a contaminant of heroin-like street drugs)
|
 |
While some neurotoxic agents affect neurons throughout the body, others are quite selective. For example, methanol specifically affects the optic nerve, retina and related ganglion cells. Trimethyltin kills neurons in the hippocampus, a region of the cerebrum. MPTP produces a "Parkinson-like disease" by damaging neurons in an area of the midbrain (substantia nigra).
Other agents can degrade neuronal cell function by diminishing its ability to synthesize protein, which is required for the normal function of the neuron. Organomercury compounds exert their toxic effect in this manner.
With some toxins, only a portion of the neuron is affected. If the cell body is killed, the entire neuron will die. Some toxins can cause death or loss of only a portion of the dendrites or axon while the cell itself survives but with diminished or total loss of function. Commonly axons begin to die at the very distal end of the axon with necrosis slowly progressing toward the cell body. This is referred to as "dying-back neuropathy".
Some organophosphate chemicals (including some pesticides) cause this distal axonopathy. The mechanism for the dying back is not clear but may be related to the inhibition of an enzyme (neurotoxic esterase) within the axon. Other well known chemicals can cause distal axonopathy include ethanol, carbon disulfide, arsenic, ethylene glycol (in antifreeze) and acrylamide.
Interference with Electrical Transmission: There are two basic ways that a foreign chemical can interrupt or interfere with the propagation of the electrical potential (impulse) down the axon to the synaptic junction. One is to interfere with the movement of the action potential down the intact axon. The other mechanism is to cause structural damage to the axon or its myelin coating. Without an intact axon, transmission of the electrical potential is not possible.
Interruption of the propagation of the electrical potential is caused by agents that can block or interfere with the sodium and potassium channels and sodium-potassium pump. This will weaken, slow, or completely interrupt the movement of the electrical potential. Many potent neurotoxins exert their toxicity by this mechanism.
Tetrodotoxin (a toxin in frogs, puffer fish and other invertebrates) and saxitoxin (a cause of shellfish poisoning) blocks sodium channels. Batrachotoxin (toxin in South American frogs used as arrow poison) and some pesticides (DDT and pyrethroids) increases the permeability of the neuron membrane preventing closure of sodium channels which leads to repetitive firing of the electrical charge and an exaggerated impulse.
A number of chemicals can cause demyelination. Many axons (especially the PNS) are wrapped with a protective myelin sheath which acts as insulation and restricts the electrical impulse within the axon. Agents that selectively damage these coverings disrupt or interrupt the conduction of high-speed neuronal impulses. Loss of a portion of the myelin can allow the electrical impulse to leak out into the tissue surrounding the neuron so that the pulse does not reach the synapse with the intended intensity.
In some diseases, such as Multiple Sclerosis and Lou Gehrig's Disease (Amyotrophic Lateral Sclerosis) the myelin is lost causing paralysis and loss of sensory and motor function. A number of chemicals are known to cause demyelination. Diphtheria toxin causes loss of myelin by interfering with the production of protein by the Schwann cells that produce and maintain myelin in the PNS. Triethyltin (used as a biocide, preservative, and polymer stabilizer) interrupts the myelin sheath around peripheral nerves. Lead causes loss of myelin primarily around peripheral motor axons.
Interference with Chemical Neurotransmission: Synaptic dysfunction is a common mechanism for the toxicity of a wide variety of chemicals. There are two types of synapses, those between two neurons (axon of one neuron and dendrites of another) and those between a neuron and a muscle cell or gland. The basic mechanism for the chemical transmission is the same. The major difference is that the neurotransmitting chemical between a neuron and muscle cell is acetylcholine whereas there are several other types of neurotransmitting chemicals involved between neurons, depending on where in the nervous system the synapse is located.
There are four basic steps involved in neurotransmission at the synapse:
 |
 |
 |
|  | Synthesis and storage of neurotransmitter (synaptic knob of axon)
|
 |
|  | Release of the neurotransmitter (synaptic knob with movement across synaptic cleft)
|
 |
|  | Receptor activation (effector membrane)
|
 |
|  | Inactivation of the transmitter (enzyme breaks down neurotransmitter stopping induction of action potential)
|
 |
The arrival of the action potential at the synaptic knob initiates a series of events culminating in the release of the chemical neurotransmitter from its storage depots in vesicles. After the neurotransmitter diffuses across the synaptic cleft it complexes with a receptor (membrane-bound macromolecule) on the post-synaptic side. This binding causes an ion channel to open, changing the membrane potential of the post-synaptic neuron or muscle or gland. This starts the process of impulse formation or action potential in the next neuron or receptor cell. However, unless this receptor-transmitter complex is inactivated, the channel remains open with continued pulsing. Thus, the transmitter action must be terminated. This is accomplished by specific enzymes that can break the bond and return the receptor-membrane to its resting state.
Drugs and environmental chemicals can interact at specific points in this process to change the neurotransmission. Depending on where and how the xenobiotics act, the result may be either an increase or a decrease in neurotransmission. Many drugs (such as tranquilizers, sedatives, stimulants, beta-blockers) are used to correct imbalances to neurotransmissions (such as occurs in depression, anxiety, and cardiac muscular weakness). The mode of action of some analgesics is to block receptors, which prevent transmission of pain sensations to the brain. There are many other situations in which drugs are used to modify neurotransmission.
Exposure to environmental chemicals that can perturb neurotransmission is a very important area of toxicology. Generally neurotoxins affecting neurotransmission act to increase or decrease the release of a neurotransmitter at the presynaptic membrane, block receptors at the postsynaptic membrane, or modify the inactivation of the neurotransmitter.
The list of neurotoxins is long and varied so that only a few examples to show the range of mechanisms is presented:
 |
 |
 |
|  | b-Bungarotoxin (a potent venom of elapid snakes) prevents the release of neurotransmitters
|
 |
|  | Scorpion venom potentiates the release of a neurotransmitter (acetylcholine)
|
 |
|  | Black widow spider venom causes an explosive release of neurotransmitters
|
 |
|  | Botulinum toxin blocks the release of acetylcholine at neuromuscular junctions
|
 |
|  | Atropine blocks acetylcholine receptors
|
 |
|  | Strychnine inhibits the neurotransmitter glycine at postsynaptic sites resulting in an increased level of neuronal excitability in the CNS.
|
 |
|  | Nicotine binds to certain cholinergic receptors
|
 |
A particularly important type of neurotoxicity is the inhibition of acetylcholinesterase. The specific function of acetylcholinesterase is to stop the action of acetylcholine once it has bound to a receptor and initiated the action potential in the second nerve or at the neuro-muscular or glandular junction. If the acetylcholine-receptor complex is not inactivated, continual stimulation will result leading to paralysis and death.
Many commonly used chemicals, especially organophosphate and carbamate pesticides poison mammals by this mechanism. The major military nerve gases are also cholinesterase inhibitors. Acetylcholine is a common neurotransmitter. It is responsible for transmission at all neuromuscular and glandular junctions as well as many synapses within the CNS.
The complexity of the sequence of events that takes place at a typical cholinergic synapse is indicated below:
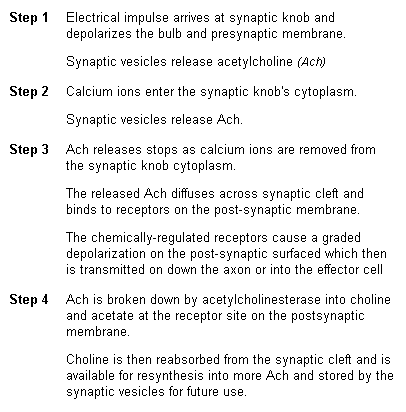
The nervous system is the most complex system of the body. There are still many gaps in the understanding as to how many neurotoxins act. On a weight basis, the most potent toxins are neurotoxins with extremely minute amounts sufficient to cause death.

  
|
|
|
|